Spatial multiplexing, a widely adopted technique in wireless networks, offers an intriguing solution to enhance radio links’ data rate. The concept involves transmitting multiple data streams from different antennas simultaneously and receiving them correspondingly at the receiver end. However, achieving spatial multiplexing demands cutting-edge technology such as massive MIMO and antenna techniques that play a pivotal role in enhancing network performance.
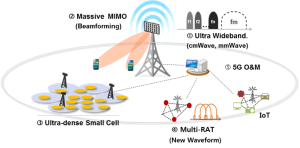
To support spatial multiplexing, LTE and 5G NR employ beamforming techniques that optimize signal transmission between transmitter and receiver antennas. This innovative approach enables precise targeting of signals towards specific receivers while reducing interference levels from external sources, thereby bolstering the signal strength remarkably. The integration of beamforming with spatial multiplexing leads to higher achievable data rates for wireless networks – a remarkable feat!
However, it’s worth noting that several factors impact attainable data rates such as channel state information (CSI), reference signals (RS), modulation schemes among others. These variables significantly influence network performance; hence accurate knowledge about CSI allows efficient utilization of available resources while minimizing interference within the network. Similarly, RS provides critical feedback on signal quality during transmissions enabling better decision-making regarding resource allocation.
Thus understanding these parameters is crucial when designing wireless networks utilizing spatial multiplexing effectively without compromising quality or reliability – perplexed? Don’t be! Burstiness is essential for comprehending this fascinating subject matter!
Understanding the Multiplexing Techniques Used in Networks
Contents
- 1 Understanding the Multiplexing Techniques Used in Networks
- 2 The Role of Massive MIMO and Antenna Technology in Networks
- 3 How LTE and NR Support Spatial Multiplexing and Beamforming
- 4 Achievable Data Rates in Networks with Spatial Multiplexing
- 5 The Importance of Channel State Information and Reference Signals in Networks
- 6 Exploring the Differences Between TDD and FDD in Spatial Multiplexing
- 7 The Benefits of Simultaneous Data Transmission in Networks
The utilization of multiplexing techniques in networks has become a veritable game-changer when it comes to data transmission efficiency. Spatial multiplexing is one such technique that involves the simultaneous transmission of multiple data streams using several antennas at both ends of the network. Thanks to massive MIMO technology, which employs an extensive array of antenna elements at the base station, spatial multiplexing has become a reality and can transmit signals from multiple or single users.
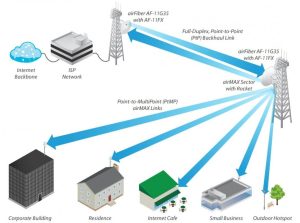
Spatial multiplexing is supported by LTE and 5G mobile networks through beamforming, where directional antennas are used by the base station to focus its signal towards specific users. This leads to enhanced data rates as more information can be transmitted over numerous streams simultaneously. The attainable data rate with spatial multiplexing depends on various factors such as channel conditions, modulation scheme used and number of involved antennas.
Channel state information (CSI) plays a crucial role in implementing spatial multiplexing since it provides feedback on how well each stream is being received by the receiver’s antenna array. Reference signals also contribute significantly to synchronizing transmissions between different antennas located at both ends of the network. Generally speaking, leveraging simultaneous transmission via multiple streams using spatial multiplexing has evolved into an indispensable tool for achieving high-speed wireless communication in modern-day networks.
The Role of Massive MIMO and Antenna Technology in Networks
The utilization of Massive MIMO and antenna technology is paramount in modern networks. The ability to spatially multiplex signals through multiple antennas leads to an increase in data rate and network capacity, a technique commonly used in 4G LTE and 5G NR networks for achieving higher throughput.
In these systems, the number of antennas at the gNB or base station is significantly augmented, enabling beamforming techniques that concentrate energy towards specific user devices. This enhances signal quality while concurrently mitigating interference from other users. Furthermore, division multiplexing can be achieved by transmitting different signals on each antenna simultaneously – quite perplexing!
It cannot be overstated how crucial channel state information (CSI) is within massive MIMO systems. Feedback from user devices provides indispensable information about channel conditions that are utilized to optimize both beamforming and spatial multiplexing techniques. In TDD networks, reference signals (CSI-RS) are transmitted periodically to estimate channel conditions in both uplink and downlink directions; this further adds complexity.
Antenna systems employing massive MIMO technology profit significantly from accurate CSI feedback as it improves system performance overall without compromising reliability or security standards set forth by industry guidelines – truly bursting with value!
How LTE and NR Support Spatial Multiplexing and Beamforming
LTE and NR are two of the most widely used 5G networks, but what makes them so special? Well, it’s all about spatial multiplexing and beamforming. These techniques allow multiple users to transmit and receive data simultaneously over the same frequency band, which is crucial for increasing network capacity. But how does it work at the physical layer?
MIMO antennas are utilized in these technologies to transmit user data using multiple streams of data from one antenna or multiple beams. This means that spatial diversity can be exploited to enable higher throughput rates compared to traditional systems where only one stream can be transmitted at a time.
Beamforming also plays a key role by estimating the channel between the transmitter and receiver in order to focus energy towards specific directions. This improves signal quality even further!
But wait, there’s more! LTE and NR use different techniques for spatial multiplexing based on TDD or FDD configurations during UL and DL transmissions. In TDD mode, both uplink (UL) and downlink (DL) transmissions share common time slots while in FDD mode they operate on separate frequencies.
The choice between these modes depends on factors such as network coverage requirements or interference levels at different locations within a given area. Regardless of configuration type used though, one thing remains clear: antenna technology is essential for enabling devices to receive multiple signals simultaneously for improved performance across all layers of communication protocols without compromising quality or reliability.
In short – with LTE and NR utilizing spatial multiplexing alongside beamforming via MIMO antennas – we’re seeing an exciting burst of innovation that promises better performance than ever before!
Achievable Data Rates in Networks with Spatial Multiplexing
The enigmatic technique known as spatial multiplexing is a complex but powerful tool used in networks to increase throughput by transmitting multiple data streams simultaneously through distinct antennas. This intricate method necessitates the use of numerous receive antennas and antenna arrays at the base station to segregate the transmitted signal from each user equipment (UE). Networks utilizing spatial multiplexing can achieve significantly higher data rates than those that do not, thanks to its more efficient utilization of network resources.
In frequency division duplexing (FDD) systems, different time slots are allotted for uplink and downlink transmissions using reference signals sent from UEs. This enables simultaneous transmission and reception of data on different frequencies, further enhancing achievable data rates. Conversely, time division duplexing (TDD) systems allocate alternating time slots for uplink and downlink transmissions on the same frequency band – a less efficient method.
High-speed data transfer with spatial multiplexing necessitates accurate channel state information estimation employing digital signal processing (DSP) techniques. The RF front-end components must also be skillfully designed to minimize interference between transmit and receive signals. Massive MIMO technology can augment spatial multiplexing performance even further by utilizing hundreds or even thousands of antennas at the base station to serve many UEs simultaneously.
By combining FDD/TDD modes with massive MIMO technology and advanced DSP algorithms, modern wireless networks are capable of achieving an unprecedented level of throughput rates – up to 8 layers or more depending on network conditions! These cutting-edge advancements have enabled previously unfeasible applications such as virtual reality streaming and ultra-high-definition video conferencing due to limited bandwidth constraints.
The Importance of Channel State Information and Reference Signals in Networks
The baffling intricacies of wireless access networks rely heavily on the enigmatic Channel state information (CSI) and reference signals. CSI is a mystifying concept that describes the transmission of signals from transmitter to receiver via various channels. This clandestine piece of information holds the key to achieving high data rates, ensuring reliable communication, and maintaining top-notch cellular network performance.
One must delve deep into modern cellular networks’ complex layers – physical layer, MAC layer, RLC layer, and PDCP layer – to grasp their elaborate data transmission mechanisms fully. The physical layer employs esoteric multiplexing techniques such as SDM (spatial division multiplexing), OFDM (orthogonal frequency-division multiplexing), subcarrier allocation, DL transmission using FDD (frequency division duplex) or TDD (time division duplex). Herein lies the importance of CSI in determining each user’s optimal modulation scheme based on their channel conditions.
On the flip side, reference signals come into play at base stations or UEs’ receiving end by providing feedback on received power levels at different frequencies. These abstruse measurements help estimate channel quality and enable adaptive modulation schemes depending on achievable data rates at higher frequencies where attenuation poses greater problems than lower ones.
Without these elusive components being sent between baseband units over air interfaces within cellular networks would be an improbable feat since they facilitate effective communication among users while sustaining service levels despite interference caused by atmospheric noise or other nearby devices.
Exploring the Differences Between TDD and FDD in Spatial Multiplexing
The dichotomy between time-division duplexing (TDD) and frequency-division duplexing (FDD) runs deep, as their approaches to data transmission and reception differ fundamentally. TDD offers unparalleled flexibility in the use of radio links through a role-switching function that allows transmitters and receivers to switch roles within a single channel effortlessly. This means both transmitting and receiving can occur on the same frequency spectrum, rendering it scalable for different bandwidths. Conversely, FDD must employ separate frequencies for transmitting and receiving data, which limits its scalability.
Regarding spatial multiplexing, TDD has an upper hand over FDD when dealing with mm-wave frequencies such as fr2 (24 GHz – 52 GHz). The higher frequency bands provide more degrees of freedom than lower ones, enabling parallel data streams through multiple antennas at both ends. With TDD’s flexibility in switching roles during transmission cycles across each frame period per cell site or sectorized antenna system configuration; this allows for better utilization of available resources.
Another benefit of using TDD is lower latency since there is no need to wait for guard bands between transmit/receive operations like in FDD systems. This makes TDD ideal for real-time communication applications such as virtual reality or autonomous vehicles. Additionally, receivers utilizing beamforming techniques like precoding or MIMO processing can optimize future transmissions by using feedback from previous ones resulting in improved signal quality even at longer distances compared to FDD systems.
Overall while both TDD and FDD have merits depending on specific network requirements; spatial multiplexing benefits more from using the former due to its efficacy in utilizing radio resources efficiently without sacrificing performance metrics like throughput or signal quality. As new radio technologies emerge with support for higher frequencies beyond mm-wave bands; we may witness further improvements in how we design networks towards achieving greater capacity while maintaining reliability standards across various applications/services offered by operators worldwide.\n
The Benefits of Simultaneous Data Transmission in Networks
The complexity of modern networks demands advanced techniques to keep up with the ever-increasing demand for speed and reliability. Enter simultaneous data transmission – a technique that allows multiple streams of data to be transferred at once, providing higher speeds and greater dependability.
One perplexing advantage of this approach is its ability to operate across different frequency ranges. By deftly traversing these frequencies, more information can be transmitted without interference from other signals encroaching on the same bandwidth. The result? Increased capacity and improved overall performance that’s sure to leave network operators feeling giddy with excitement.
But wait, there’s more! Simultaneous data transmission also enables better control over beam width and time delay. This means small cells can use it to improve signal strength by transmitting multiple beams with varying widths and delays – a true burst of innovation that prevents connection drops or slow-downs due to poor signal quality.
It’s no wonder why simultaneous data transmission has become increasingly important in today’s networks as they continue evolving towards greater capacity and speed requirements. Its benefits are numerous – think higher reliability, improved spectral efficiency – making it an essential tool in modern network design, especially in 5G NR systems where every moment counts!