Even as some schools and engineers embrace generalist status, others must specialize. Why? Simple: who creates those neatly packaged abstractions that project managers assemble into finished systems at the click of a mouse? Other EEs, of course, working as module designers and programmers. These engineers must focus on the minutiae of a particular subdiscipline—say, the timing characteristics of a particular family of CMOS chip-fabrication processes or the design of a special class of databases. Then comes the hard part: packaging their knowledge in a form that nonspecialists can use without worrying about all the details.
Ironically, as the visible face of circuits becomes more and more digital, their analog foundation becomes more and more apparent. As circuit features shrink below 100 nanometers, the quaint design-rule abstractions that allowed engineers for the most part to leave aside leakage current, parasitic capacitance, and other messy real-world issues no longer hold, says Riordan. Anyone who designs systems that operate at high speed and low power in this nano domain must know quantum field theory and solid-state physics as well as algorithms. And module builders have to work harder to maintain the digital behavior.
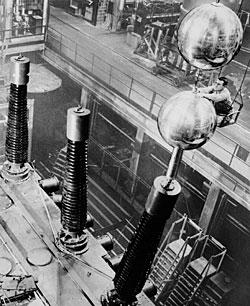
Such difficulties point to the downside of the entrenched reliance on packages, encapsulated expertise, and abstraction. Many observers have begun to worry that EEs reared on abstraction and on computer simulations that simply parrot abstract models may lose touch with the behavior of real devices. Fred G. Martin, a longtime MIT Media Laboratory researcher who is now an assistant professor at the University of Massachusetts-Lowell, tells a story of just how brittle abstract knowledge can be. One of his students complained about Martin’s lecture on transistors, fixated on a rule he had learned in a previous course, namely that collector current equals base current multiplied by gain.
As many EEs have discovered, at some point this rule is trumped by Ohm’s Law, which tells you how much current flows through a circuit with a given resistance and input voltage. But the student, who had never built a real, working circuit, was ready to believe that Martin’s discussion of Ohm’s Law was wrong because it conflicted with the shorthand rule he’d been taught about idealized transistors. A working EE would never make such a simple error.
Bert Sutherland, who retired in 2000 as director of Sun Microsystems Laboratory after a career that also included stints managing researchers at Xerox Palo Alto Research Center, expresses another concern about where the increasing reliance on modeling and simulation may be taking engineers. Sure, Sutherland says, growing computer power makes it easier to model phenomena that are already easy to model, but “things that are difficult to model stay difficult.”
As the tools themselves become more complex, the temptation to avoid approaches that aren’t amenable to existing software may increase. Techniques such as asynchronous logic or adiabatic clock distribution (in which resonant circuits recapture much of the energy usually dissipated in sending clock pulses across a chip) offer significant improvements in performance or power consumption, for example, but the chips are much harder to analyze than ones in which the gates are synchronized and all of a clock network’s energy is dissipated to ground.
The same advances that led EEs to build more complex systems also allow smaller teams—maybe even a single engineer—to handle projects that in previous decades would have called for a hangar full of men with slide rules, pocket protectors, and narrow ties. This increase in productivity poses a conundrum, says Sutherland: you have to hope that the number of projects calling for engineering talent outpaces the rate at which EEs encapsulate and standardize their knowledge, making fewer of them necessary for any given project.
MIT’s Williams points to the long-term decline in U.S. students choosing engineering as a sign that young people do not see it as a secure, comfortable career [see sidebar, “Stay Current, Stay Lucky, Stay Employed”]. Between 1987 and 2001, the U.S. Department of Education reports, the number of electrical engineering bachelor’s degrees in the United States decreased by more than 45 percent.
With EE enrollment, employment, and subject matter all in upheaval, companies and educational institutions will have to make significant adjustments. Some of them may prosper beyond expectations; others will not survive.
Wulf is optimistic: he points to the rapid revamping of curricula shortly after World War II, when EEs built a science-based educational system that effectively reclaimed their field from the physicists, who had made so many key technological advances during the war. Wulf also thinks that knowing something about electrical engineering can benefit people in other disciplines. For example, he says, a civil engineer should know enough about digital design to be able to specify how a bucketload of radio-frequency-enabled strain gauges can be installed in a bridge to let the structure diagnose itself.
For the EEs who keep up with the pace of innovation, the ride ahead will be thrilling. Quantum-based cryptographic devices are already reaching market, and their computing progeny—which in theory could simultaneously calculate all possible answers to some questions—are inching into existence in laboratories around the globe. On the biology side, EEs like Tom Knight, senior research scientist at the MIT Artificial Intelligence Laboratory, are applying principles that worked for chip-design rules and the very large-scale integration (VLSI) revolution to create stripped-down microorganisms that could be bred to lay down patterns for ultrasmall circuits made of silicon, or whatever material comes next.
Indeed, biology will reshape electrical engineering in ways we can’t imagine. Neural networks, genetic programming, computer viruses—each of these took inspiration from biological phenomena, points out Kenneth R. Foster, a professor of bioengineering at the University of Pennsylvania, in Philadelphia.
“During the span of my own career, a new discipline, bioengineering, emerged from electrical engineering and other classical engineering fields and has taken off in the directions of tissue engineering, genetics, proteomics, and neuroscience,” he says. What Foster refers to as “the spectacular science in these fields” will reshape the way that electrical engineering is practiced. For example, EEs are borrowing techniques from the world of molecular biology to assemble structures that can be used as displays or switches, and to simulate neurons.
“We are using VLSI chips to simulate the action of neurons and other biological cells,” explains Foster’s colleague Kwabena Boahen, an associate professor in the Penn bioengineering department. Boahen points out that a basic analog circuit-design modeling program such as Spice can simulate neurons “just fine.”
More EEs are getting involved with neurobiology, Boahen notes, and biologists are happy to work with them. “The biologists determine what the inner workings of a neuron are,” he explains. “They tell what the pieces are, and I’ll design a circuit to mimic how these pieces work together.” Boahen himself is a prime example of an EE who migrated to biology. He holds bachelor’s and master’s degrees in electrical and computer engineering and a doctorate in computation and neural systems.
So in such a complex new industrial and educational ecology, how will we recognize EEs? By their stance, Riordan says: feet squarely planted on terra firma, but with a gaze out toward the horizon. Musing about why he became an EE, he cheerfully concedes that he didn’t crave pure intellectual exploration, as the best scientists do. Nor did he have whatever turn of brain it takes to make fine art. What he had then, and has now, is “the specific ability to deal with the real world as it exists and craft things from it.” Others with similar talents will find in electrical engineering a heady lifelong stimulus. Riordan concludes, “I can’t think of a more fortunate path than the one I followed.”
About the Author
PAUL WALLICH is a science writer who lives in Montpelier, Vt.
To Probe Further
Two histories of electrical engineering were published by the IEEE Press in 1984. One of the books, Engineers & Electrons, by John D. Ryder and Donald G. Fink, was a breezy overview aimed at a broad readership. The other, The Making of a Profession: A Century of Electrical Engineering in America, was a more scholarly volume written by A. Michal McMahon.
Rosalind H. Williams of the Massachusetts Institute of Technology incorporated personal reminiscences and family history into her book Retooling: A Historian Confronts Technological Change (MIT Press, 2002).